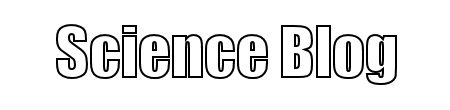
April
2002
From New Scientist
Artificial organs
Researchers have high hopes of building an artificial
liver
THE man who grew a human ear on the back of a mouse has
made a breakthrough that brings the prospect of an artificial
liver much closer. He believes he has solved the problem of
growing the complex networks of blood vessels that artificial
organs would need to sustain themselves within the body.
Until now, researchers trying to build replacement body
parts have been limited to making relatively simple tissue,
such as thin sections of cartilage and skin. That's because it
has not been possible to grow the deep networks of blood
vessels that organs need to stay alive. But now Jay Vacanti at
Massachusetts General Hospital in Boston and Jeffrey
Borenstein at the nearby Draper Lab have shown that it can be
done.
Artificial organs would be a boon because organ donations
cannot keep pace with demand. In the US, some 80,000 people
are waiting for kidney, liver or heart transplants. And of the
23,000 Americans who need a liver, only 5000 got their wish
last year. Artificial organs would slash these waiting lists
and solve the big problem of organ rejection by growing them
from the patient'scells.
Tissue engineers can already grow simple body tissues such
as knee cartilage. They start with a shaped, porous scaffold
made from a biodegradable plastic like polylactic glycolic
acid (PLGA). The scaffold is "seeded" by plunging it into a
solution of the patient's cells and then immersing it in a
nutrient solution. As the cells multiply and clump together,
the scaffold dissolves, leaving a piece of cartilage ready for
transplant.
The new cartilage can survive because oxygen and nutrients
from surrounding body fluids diffuse into the cells. But this
doesn't work for the thicker tissues typical of livers and
kidneys because nutrients can only diffuse across a few cell
layers. Instead, they need an internal blood supply to deliver
nutrients to cells deep inside the organ. "Without a blood
supply, the tissue dies," says Borenstein, a micro-engineering
expert.
In a bid to beat the problem, Borenstein teamed up with
transplant surgeon Vacanti, who in 1997 famously grew a human
ear from cartilage cells on the back of a mouse. The idea the
pair hatched involves copying the blood vessel network of a
real liver and using 3D computer modelling and machining to
mimic its construction.
First, they injected a liquid plastic into the blood
vessels of a liver. Once the plastic had solidified, they
dissolved the liver tissue, leaving a solid cast of the
organ's blood vessels. From this model they were able to take
measurements of vessel diameters, branching angles and the
distances between vessels. They fed the numbers into software
that built a 3D fractal-like computer model of a liver's blood
supply. When they simulated blood flowing through the "liver",
it had the same blood flow properties as the living system,
says Borenstein.
The 3D blood vessel model is then "sliced up" inside the
computer, dividing the model into a series of horizontal
layers, each of which is used to make a silicon mould. PLGA is
then poured into each mould to make the many slices that when
sandwiched together, under pressure and heat, create a
scaffold for the artificial liver. It's shot through with
channels as small as the finest capillaries and as large as
the organ's main veins and arteries.
The scaffold then has to be seeded with the cells that make
up the solid part of the liver. As well as hepatocytes, which
perform key liver functions such as breaking down toxins and
metabolising proteins and carbohydrates, there are at least
six other types of cells. Borenstein says there are two
possible ways of seeding this complex network of different
cell types. You could seed each layer separately with
different cell types, or inject them from the outside. But the
pair won't yet say how this is done.
To get the blood supply working, a solution of endothelial
cells, the cells that normally line blood vessels, is pumped
into the empty channels in the scaffold, where they stick to
the walls. These cells grow in a nutrient to form a network of
blood vessels within the scaffold which itself dissolves over
a few months, leaving behind a functioning liver-at least,
that's the aim. So far, only blood vessel networks grown this
way have been tested in rats, with no leakage or obstruction
of the blood flow.
This is still a long way from a working liver. Linda
Griffith, a tissue engineer at MIT, says getting all the right
cell types to grow in the right places in the "liver" is
crucial. "In just a gram of liver, you have around 100 million
cells and it'd be very hard to position each and every one,"
she says, suggesting that encouraging smaller numbers of
different types of liver cells to self-assemble may help.
But there's another enemy waiting in the wings. "Currently,
there's no way of keeping these implants sterile, and no one
is really looking at that," warns tissue engineer Larry Hench
at London's Imperial College. Bacteria love the warm nutrient
solutions and would spread quickly. "If we can't sort this
problem out, we could be stuck with lab-based solutions we
can't use," he warns.
Author: Ian Sample
UK CONTACT - Claire Bowles, New Scientist Press Office,
London: Tel: +44(0)20 7331 2751 or email claire.bowles@rbi.co.uk
New Scientist issue: 27 APRIL 2002
PLEASE MENTION NEW SCIENTIST AS THE SOURCE OF THIS STORY
AND, IF PUBLISHING ONLINE, PLEASE CARRY A HYPERLINK TO: http://www.newscientist.com/
"These articles are posted on this site to give advance
access to other authorised media who may wish to quote
extracts as part of fair dealing with this copyrighted
material. Full attribution is required, and if publishing
online a link to http://www.newscientist.com/
is also required. Advance permission is required before any
and every reproduction of each article in full - please
contact angela.bourton@rbi.co.uk.
Please note that all material is copyright of Reed Business
Information Limited and we reserve the right to take such
action as we consider appropriate to protect such copyright."
|